Abstract
BACKGROUND: The novel coronavirus-associated ARDS (COVID-19 ARDS) often requires invasive mechanical ventilation. A spectrum of atypical ARDS with different phenotypes (high vs low static compliance) has been hypothesized in COVID-19.
METHODS: We conducted a retrospective analysis to identify respiratory mechanics in COVID-19 ARDS. Berlin definition was used to categorize severity of ARDS. Correlational analysis using t test, chi-square test, ANOVA test, and Pearson correlation was used to identify relationship between subject variables and respiratory mechanics. The primary outcome was duration of mechanical ventilation. Secondary outcomes were correlation between fluid status, C- reactive protein, PEEP, and D-dimer with respiratory and ventilatory parameters.
RESULTS: Median age in our cohort was 60.5 y with predominantly male subjects. Up to 53% subjects were classified as severe ARDS (median = 86) with predominantly low static compliance (median Cst- 25.5 mL/cm H2O). The overall mortality in our cohort was 61%. The total duration of mechanical ventilation was 35 d in survivors and 14 d in nonsurvivors. High PEEP (r = 0.45, P < .001) and D-dimer > 2,000 ng/dL (P = .009) correlated with significant increase in physiologic dead space without significant correlation with
. Higher net fluid balance was inversely related to static compliance (r = −0.24, P = .045), and elevation in C- reactive protein was inversely related to
(r = −0.32, P = .02).
CONCLUSIONS: In our cohort of mechanically ventilated COVID-19 ARDS subjects, high PEEP and D-dimer were associated with increase in physiologic dead space without significant effect on oxygenation, raising the question of potential microvascular dysfunction.
QUICK LOOK
Current Knowledge
ARDS due to novel coronavirus 2019 is associated with high mortality. Lung-protective mechanical ventilation is associated with improved mortality in ARDS patients, and use of high PEEP is recommended for optimal oxygenation. However, no clear clinical marker has been identified to titrate PEEP for individualized care, and elevated PEEP can lead to increased dead-space ventilation and subsequent clinical deterioration.
What This Paper Contributes to Our Knowledge
We identified that high PEEP was associated with increased physiologic dead space without significant effect on oxygenation. Our study demonstrates the association of D-dimer (a marker of vascular injury and thrombosis) with increased dead-space ventilation, raising the question of pulmonary microvascular dysfunction in COVID-19 ARDS.
Introduction
The coronavirus disease 2019 (COVID-19) caused by the novel coronavirus (SARS-CoV-2) was declared a global pandemic by World Health Organization (WHO) and has affected over 7.2 million people, with over 413,372 deaths to date.1,2 ARDS is a severe respiratory manifestation of COVID-19, often requiring admission to the ICU and invasive mechanical ventilation. While fulfilling the Berlin definition of ARDS,3 some authors believe COVID-19 ARDS represents an unfamiliar presentation of the syndrome.4 It has been postulated that microvascular injury leading to dysregulation of lung perfusion and hypoxic vasoconstriction could explain the discordance in lung compliance and severity of hypoxemia.4 However, pulmonary vascular dysfunction has also been described in “usual” ARDS as a potential explanation of worsening ventilation-perfusion (V̇/Q̇) mismatch and hypoxemia.5 Hypercapnia and dead space (VD/VT) is also a concern in ARDS, with studies showing that worsening hypercapnia or increased VD/VT is a risk factor for mortality in these subjects.6,7
In this study, we aimed to study a subset of subjects admitted to our ICU with confirmed COVID-19 ARDS and describe clinical characteristics, lung mechanics, correlation with inflammatory markers, and various treatment strategies to manage ARDS.
Methods
Study Design and Participants
We conducted a retrospective observational analysis of subjects admitted to the NYC Health + Hospitals/Bellevue Hospital medical ICU between March 10, 2020, and April 7, 2020, with COVID-19 confirmed by SARS-CoV-2 reverse transcription-polymerase chain reaction (RT-PCR) and respiratory failure requiring invasive mechanical ventilation. The study was approved by the New York University School of Medicine Institutional Review Board (IRB # i20-00447) and NYC Health + Hospitals system to track and report, and a waiver of informed consent was granted due to the observational nature of the study.
Data Collection and Definitions
A subcohort of 26 subjects was identified based on the existence of specific ventilator-related data. Subject demographics and clinical data were extracted from electronic medical records. Ventilator settings including peak airway pressures, PEEP, plateau pressure (Pplat), tidal volume (VT), and breathing frequency were manually collected during medical care. Pplat was measured for all subjects through a brief inspiratory hold maneuver. All data were entered into REDCap, a secure, web-based software platform designed to support data capture for research studies, hosted at New York University Langone Health.8,9
ARDS and its severity were defined using the Berlin definition established by the ARDS Definition Task Force.3 Predicted body weight (PBW) calculations were done according to ARDSNet protocol. Along with PBW, , driving pressure (ΔP), static compliance (Cst), and ventilatory ratio (VR), an index of ventilation efficiency that correlates well with physiologic VD,10,11 were calculated using the equations provided in Table 1.
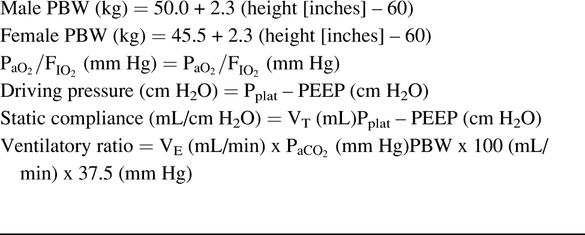
Respiratory Mechanics Equations
The primary outcome was duration of invasive mechanical ventilation. Follow-up time was right censored on May 18, 2020. Secondary outcomes included correlation of fluid status, PEEP, and inflammatory marker levels with respiratory and ventilator parameters. Other outcomes included time from presentation to initiation of mechanical ventilation, need for tracheostomy, and mortality.
Statistical Analysis
Continuous variables were expressed as median with interquartile range (IQR). Categorical variables were expressed as n (%). Missing values were not imputed. Differences in frequencies were analyzed using the chi-square test or Fisher exact test. Differences in means or medians were analyzed using independent t test or Wilcoxon-Mann-Whitney test. Analysis of variance (ANOVA) was used to compare multiple groups. Pearson correlation (R) and its 95% CI were estimated to quantify the correlation between 2 variables. Analysis was performed using R programming language (version 3.4.4, R Foundation).12-14
Results
Demographics and General Outcomes
The demographic characteristics and general clinical outcomes of our cohort are outlined in Table 2. The cohort was male predominant (n = 22/26, 84.6%) with a median age of 60.5 y. The median body mass index was 27.85 kg/m2 (IQR 27.11, 33.34). Major medical comorbidities included hypertension (53.8%) and diabetes mellitus (50%). The median time from initial hospital presentation to intubation was 2 d, with a median of 3 d in survivors compared to 1 d in nonsurvivors (P = .039). The median at the time of intubation was 86 mm Hg (IQR 65.5, 143.38) with no significant difference between survivors and nonsurvivors. Most cases met criteria for severe ARDS at intubation (n = 14/26, 60.9%). The total duration of mechanical ventilation was 35 d in survivors and 14 d in nonsurvivors. Use of neuromuscular blockade was marginally higher in nonsurvivors (93.8%, n = 15/16) compared to survivors (80%, n = 8/10), but the analysis did not reach statistical significance (P = .66). Of the 26 subjects, 2 were successfully extubated and 10 underwent tracheostomy (8 in survivors compared to 2 in nonsurvivors, P = .002). At the time of analysis, the overall ICU mortality in our cohort was 61.5% (n = 16/26).
Subject Characteristics and General Outcomes
Respiratory and Ventilator Parameters and Measurements
Table 3 summarizes the various observations made during the course of mechanical ventilation of the cohort. Compared to observations of > 200 mm Hg,
lower than 200 mm Hg (consistent with moderate and severe ARDS) were associated with higher median ΔP (18 [IQR 14–23] vs 13 [IQR 10–17] cm H2O, Wilcoxon r = 0.35, P = .007, 95% CI 1.00–8.00) and lower Cst (25 [IQR 20.29–32.14] vs 33.3 [IQR 25.74–43.75] mL/cm H2O, Wilcoxon r = 0.32, P = .01, 95% CI −14.44 to −1.59).
Measured Respiratory and Ventilator Parameters
Figure 1 depicts Cst over time for the subjects whose ventilator mechanics were evaluated in the first 3 d following intubation. Figure 2 depicts Cst over time for each individual subject for whom multiple observations were available. Figure 3 depicts over time for individual subjects for whom data were available.
Static compliance of selected subjects over the first 72 h of intubation. Supine and prone body positions are indicated by upward- and downward-facing triangles, respectively. * indicates that the subject was paralyzed at the time of observation.
Changes in static compliance during mechanical ventilation in subjects who had 2 or more measurements obtained.
Changes in during mechanical ventilation in subjects who had 2 or more measurements obtained.
Figure 4 shows the correlation of PEEP with various parameters. VR was also found to be higher in subjects ventilated at a higher PEEP (r = 0.45, P < .001, 95% CI 0.23–0.64]), suggesting a positive correlation between PEEP and VD/VT. PEEP also positively correlated with higher (r = 0.33, P < .007, 95% CI 0.10–0.54]). There was correlation with increased Cst, although this did not reach statistical significance (r = 0.2, P = .09, 95% CI −0.30 to 0.42). Notably, increase in PEEP was not significantly associated with improvement in
(P = .16, 95% CI [−0.42 to 0.07]).
Effect of PEEP on parameters. A: , B: static compliance, C:
, and D: ventilatory ratio.
Figure 5 shows the correlation of and VR with C-reactive protein levels. C- reactive protein was correlated with decreased
(r = −0.32, P = .02, 95% CI −0.56 to −0.05) but not VR (P = .32, 95% CI −0.14 to 0.41]. Figure 5 also depicts median
and VR above and below a D-dimer cutoff of 2,000 ng/mL. Median VR was significantly higher at D-dimer > 2,000 ng/mL versus < 2,000 ng/mL (2.96 [IQR 2.50–3.34] vs 2.41 [IQR 1.67–2.83], Wilcoxon r = 0.36, P = .01, 95% CI −1.21 to −0.18). Figure 6 depicts correlation of Cst and the length of stay fluid balance (total fluid balance from admission to the time of data collection) in L. Higher net length of stay fluid balance was found to be inversely related to static compliance (r = −0.24, P = .045, 95% CI −0.453 to −0.006).
A: versus measured C-reactive protein level, B:
versus measured D-dimer levels > or < 2,000 ng/mL, C: ventilatory ratio versus measured C- reactive protein level, and D: ventilatory ratio versus measured D-dimer levels > or < 2,000 ng/mL.
Static compliance correlated to total stay fluid balance.
Discussion
Lung injury in COVID-19 is caused by direct viral cytopathic effect on type 2 pneumocytes leading to diffuse alveolar damage and subsequently ARDS.15 Our study included a cohort of 26 intubated, SARS-CoV-2 RT-PCR–confirmed COVID-19 ARDS subjects with the aim of understanding the respiratory mechanics and pulmonary pathophysiology of COVID-19 ARDS.
The majority of subjects requiring mechanical ventilation (53%, n = 14/26) met criteria for severe ARDS at the time of intubation. The median time from the time of hospital presentation to intubation was longer in survivors. This may suggest that either nonsurvivors presented late in their disease course or had more severe disease at presentation. Only 2 subjects were successfully extubated, and the overall mortality was 61% (n = 16/26), which is proportional to the larger ICU cohort and similar to another large study from our region.16
Mechanical Ventilation in COVID-19 ARDS
The approach to mechanical ventilation in ARDS is largely supportive in nature, revolving around mitigating lung injury while the underlying cause resolves. ARDS subjects on mechanical ventilation are at risk for ventilator-induced lung injury (VILI), which can occur by a variety of mechanisms including lung overdistention (“volutrauma”), high transpulmonary pressure (“barotrauma”), repetitive opening and closing of alveoli (“atelectrauma”), and release of pro-inflammatory mediators (“biotrauma”).17 In order to prevent VILI, the American Thoracic Society recommends adoption of a lung-protective ventilation strategy for ARDS, which emphasizes low VT ventilation at 4–8 mL/kg PBW and limitation of inspiratory pressures (Pplat < 30 cm H2O).18 Additionally, VILI is a concern in all mechanically ventilated patients, whether they have ARDS or not.19,20 While we attempted to institute lung-protective ventilation strategies, we could have been limited by ventilator asynchrony that may develop as a result of such a strategy. As a result, the median VT in our cohort was 8.99 mL/kg PBW (IQR 8.29, 9.83), median breathing frequency was 23 per min, and median V̇E was 9.9 L/min. However, in keeping with other lung-protective ventilation guidelines, the median Pplat was 26 cm H2O (IQR 22.5, 31.5).
Respiratory Mechanics in COVID-19 ARDS – Dynamic, not Dichotomous
In COVID-19 ARDS, low VT ventilation has been called into question.21 Gattinoni et al hypothesized a spectrum of disease with 2 distinct phenotypes defined by time-related differences in elastance (E) or compliance, V̇/Q̇ ratio, lung weight, and recruitability.22 However, it is likely that this merely describes a spectrum of lung compliance measurements, at par with “usual” ARDS. This is consistent with a series of COVID-19 ARDS subjects where lung mechanics have been reported.23,24 Our data also show a high degree of heterogeneity among subjects with respect to Cst, as depicted temporally in both Table 1 and Figure 1 Measured Cst in the first 24 h of mechanical ventilation ranged from 16.07–50.0 mL/cm H2O, with a median of 26.67 mL/cm H2O. In comparison, Ziehr at al reported a median static compliance 35 mL/cm H2O (IQR, 30–43).25 in our cohort also similarly varied widely, both from subject to subject and from day to day for any given subject (Fig. 2), simply reflecting the various physiologic states of a complex disease.
Positive End Expiratory Pressure in COVID-19 ARDS
The theoretical construct of high and low E phenotypes of COVID-19 ARDS was accompanied by an opinion to use higher PEEP on the high E subset of subjects.22 Our data show that increasing levels of PEEP, while not clearly associated with improved in , were significantly associated with increased
and VR, suggesting worsening physiologic dead space (Fig. 3). The implications of this phenomenon are discussed in a subsequent section. Higher PEEP levels can also paradoxically worsen hypoxemia through worsening of V̇/Q̇ matching and decreased oxygen delivery.26 This bears important implications in the implementation of guidelines for the mechanical ventilation of patients with COVID-19 ARDS, namely that routine adherence to the ARDSNet PEEP ladder27 may be fraught with challenges. Application of higher PEEP may lead to increased lung stress and worsen lung inflammation,28 thereby compounding lung injury and possibly hypoxemia as discussed below.
Lung Inflammation and Oxygenation in COVID-19 ARDS
Immune-mediated destruction of alveolar endothelium and epithelium in ARDS leads to inflammatory exudate in the alveolar space and interstitium of the lung, leading to hypoxemia due to V̇/Q̇ mismatch.29,30 C- reactive protein is a marker of systemic inflammation and has been studied as a marker of disease severity in COVID-19 pneumonia.31,32 We found that C- reactive protein levels significantly correlated with worse as a marker of oxygenation, but this same relationship was not seen with VR (Fig. 4). Whether this is indicative of the severity of the underlying disease or VILI is unclear and requires further investigation. VILI shares many pathophysiologic features with ARDS; both high tidal volumes and high PEEP increase the mechanical power delivered to the lung, thereby increasing the risk of VILI.33 Furthermore, subjects with severe COVID-19 pneumonia exhibit features of systemic hyper-inflammation or cytokine storm.34,35 Cytokines may be mechanistic for additional lung injury, markers of disease severity, and potential targets for therapy.36 While not unique to SARS-CoV-2, historically nonsurvival in ARDS has been linked to sustained interleukin (IL)-6 and IL-1 elevation.37
Dead Space and Ventilatory Efficiency in COVID-19 ARDS
In addition to the effects described above, the acute inflammatory response in COVID-19 pneumonia is associated with elevated inflammatory markers and D-dimer.36 Several reports have suggested an increased risk of thromboembolism and formation of microthrombi in different organs.38 Interestingly, most of these thrombi have been shown to be platelet mediated originating from endothelial injury by the virus.15,39 At our institution, we instituted a protocol for empiric systemic anticoagulation to be initiated for either D-dimer levels > 2,000 ng/dL or rapid doubling of D-dimer values to minimize the risk of thromboembolic disease. In this cohort, we found that subjects with D-dimer levels > 2,000 ng/dL had significantly higher VR than those with lower Ddimers (Fig. 4). This may suggest a correlation between elevated D-dimer as a marker of thrombosis and VD/VT. Systemic inflammation leads to a hypercoagulable state causing pulmonary vascular endothelial dysfunction, abnormal angiogenesis, microthrombi, and worsening V̇/Q̇ mismatch and worsening VD/VT.40,41 Microvascular injury and dysfunction as a cause of VD/VT in ARDS has previously been hypothesized.42 However, the correlation of high PEEP with increased VR in this study cannot be understated. Whereas PEEP is often thought of only for its effect on oxygenation, alveolar overdistention in compliant areas of the lung due to excessive PEEP can lead to increased VD/VT,26 which reduces ventilatory efficiency. VR correlates well with VD/VT in ARDS, and the relationship between high VD/VT or lack of improvement with mortality in ARDS is well known.6,7,11
Fluid Management
A fluid conservative strategy has been recommended in the management of ARDS patients.43 Autopsy reports in COVID-19 ARDS have demonstrated increased lung weight and extravascular fluid content that may mostly suggest increased inflammatory infiltrates but also extravascular fluid collection.15 It is imperative to monitor net input and output in ARDS patients and minimize excessive fluid therapy. Intuitively, we found that static compliance was inversely correlated to the stay fluid balance (Fig. 5). Whereas our finding reinforces the recommendation for fluid conservative management in COVID-19 ARDS, this does not necessarily extend to aggressive diuresis. This is because systemic inflammation and mechanical ventilation can lead to increased insensible losses, and hypovolemia may potentiate the effects of PEEP on both alveolar distention and cardiac output due to increased West zone 1 physiology.26,44,45
Limitations
Our study has several limitations. In the midst of the COVID-19 pandemic and significant subject volumes, we were able to collect full ventilator data in only 26 subjects that were included in our study. Whereas the small sample may limit interpretation of clinical characteristics and outcomes, we identified clear physiologic findings in COVID-19 described above. In retrospective analyses, there is a risk of measurement bias in certain variables such as net fluid balance and hemodynamics. Nevertheless, the arterial blood gas measurements and ventilator settings were recorded personally at the subject bedside by the authors of this study. Lung-protective ventilation was also not always able to be adhered to, and this may have led to more severe lung injury during mechanical ventilation. Exhaled CO2 monitoring was not consistently available; we were thus unable to calculate VD/VT using modified Bohr-Enghoff equations for our cohort. Additionally, heterogeneity in hemodynamics and critical illness may affect the total CO2 production in each individual and may not conform to established V̇E to charts that are standardized in adult patients on mechanical ventilation without ARDS.46 However, the VR described by Sinha et al scales well with ventilatory efficiency and alveolar VD, as it is anticipated that for a given individual variation in alveolar ventilation is greater than production of CO2 (
).10
Conclusions
In our cohort, we identified COVID-19 ARDS as a heterogeneous disease with variable but mostly low static compliance that followed a spectrum of physiology seen in conventional ARDS, as has been documented in the literature. We observed worsening static compliance with increasing net fluid balance as previously reported. There was no observed benefit in oxygenation or static compliance with higher PEEP. There was, however, a strong correlation of high PEEP with increased VR and .
There was a statistically significant correlation of elevated C- reactive protein with worsening hypoxemia. There was also a correlation between elevated Ddimer (> 2,000 ng/dL) and increased VR. Further research is required to determine whether these findings possibly reflect the effects of lung inflammation (either through ARDS or VILI) and the theory of microvascular injury contributing to increased VD/VT, respectively.
Through this study, we conclude that it remains intuitive and important to assess the clinical characteristics of each subject to provide optimal and physiologically targeted lung-protective ventilation, including application of PEEP. PEEP titration should be individualized and excessive PEEP avoided, as overdistention of normal alveoli may lead to worsening hypoxemia, hypercapnia, or hypotension.26 Lung-protective ventilation strategy with VT 4–8 mL/kg PBW should be emphasized in all patients to prevent VILI but may not be always feasible due to challenges with ventilation owing to asynchrony or hypercapnic respiratory acidosis.
Footnotes
- Correspondence: Himanshu Deshwal MD, Division of Pulmonary, Critical Care, and Sleep and Medicine, New York University Grossman School of Medicine, New York, New York. E-mail: himanshu.deshwal{at}gmail.com
The authors have no conflicts of interest to disclose.
- Copyright © 2021 by Daedalus Enterprises